| Interviews with Nutritional Experts: Oxygen Radicals, Pro-oxidants and Antioxidant Nutrients | |
Interview with Dr. Lester Packer as interviewed by Richard A. Passwater PhD
Professor Lester Packer, Ph.D. is the Director of the Membrane Bioenergetics Group and Professor of Molecular Biology at the University of California
at Berkeley. His distinguished career in teaching and research has included
appointments at Dartmouth Medical School and the University of Texas Southwestern
Medical School. Dr. Packer is also a Faculty Senior Scientist in the Energy
and Environment Division of the Lawrence Berkeley Laboratory.
Dr. Packer is the author of about 40 books and about 400 scientific research
articles. He is the Executive Editor of "Archives of Biochemistry and
Biophysics," and serves on the editorial boards of "Free Radical
Biology and Medicine," "Journal of Applied Nutrition" and
"Journal of Optimal Nutrition."
Dr. Packer's research interests include the role of vitamin E at the membrane
and cell level, nutritional and physiological studies in animal and human
exercise, and studies of vitamin E in the skin.
As most of you know, I have been working with free radicals since the 1960's.
At the NNFA annual meeting in Nashville, I was asked to speak on the effects
of exercise-induced free radicals and the increased need for antioxidant
nutrients. The leading researcher in this field is Professor Lester Packer,
Ph.D. of the University of California at Berkeley. I have been following
Dr. Packer's free radical research since 1971, and his free radical and
exercise research since 1984, but in June, I had the opportunity to discuss
his recent research with him in detail while horseback riding with Dr. William
Pryor and Dr. Packer in Montana.
Passwater: First of all, congratulations of receiving the
1992 VERIS Award for your significant contributions in clinical applications
of vitamin E. What got you interested in this line of research?
Packer: Well, thank you. There were a number of things that
influenced me. Looking back on it , maybe I didn't have much choice. I had
been exposed to vitamin E research and there were some interesting questions
that piqued my curiosity. For example, why was it so difficult to induce
vitamin E deficiency in adult animals and not difficult in young animals?
That was a curious thing.
I knew of the interesting antioxidant functions of vitamin E because of
the pioneering work of Dr. Al Tappel and many others. So I had that background
information. This antioxidant research was emerging at the time I was doing
mu Post Doctoral studies at the University of Pennsylvania. Dr. Al Tappel
was doing research there while on Sabbatical leave from the University of
California at Davis. I had a chance to work with him on vitamin E's antioxidant
actions, and I became interested in how vitamin E protected membranes against
damage.
Another motivating factor was the idea that vitamin E was actually discovered
at the University of California in Berkeley in this very same building in
which I was doing my research, by Dr. Herbert Evans in 1922. His legacy
was around when I came here and joined the faculty. So that was another
motivating factor.
So I became curious to work with vitamin E. There were a number of things
that happened and lines converged.
Passwater: How fortuitous for everyone. It looks like you
were pre-ordained to research vitamin E. Dr. Packer, you like to call oxygen
"our dangerous friend." Most people are aware only of the need
for oxygen. Would you elaborate on the dangerous side of the oxygen cycle?
Packer: The fact that oxygen can be toxic has been known for
a century. Pasteur killed certain organisms with oxygen. Evidence has been
building that there are toxic products from oxygen that participate in reactions
that accelerate aging and age-related diseases.
What caught my interest was that the chronic damage from oxygen toxicity
was not a direct concentration effect like that of a poison, but that the
damage was greater than what one would expect from just the number of oxygen
molecules involved. Oxygen metabolism produces side products, called oxygen
free radicals, that in turn multiply the damage of the original oxygen molecule.
These oxygen free radicals perpetuate many other free radicals setting off
a chain of damaging reactions. This damage is at the molecular and cellular
levels.
This damage, rather than being overtly toxic, is eventually expressed as
accelerated aging or diseases such as cancer, atherosclerosis or cataracts.
These species of oxygen that arise and are free to react are the so-called
oxygen free radicals. A free radical can be defined as an atom or molecule
that has one or more unpaired electrons.
Passwater: What are the most harmful oxygen radicals and how
harmful are they?
Packer: Some species of oxygen-formed free radicals are not
so dangerous, but others are more dangerous. The harm done by oxygen radicals
depends on the amounts, where they are formed and the biological system
involved.
Actually, the Oxygen molecule itself, a molecule consisting of two oxygen
atoms, is a bi-radical. But, curiously, molecular oxygen -- which is actually
two free radicals united -- is not particularly dangerous because the electronic
spins of the unpaired electrons happen to be in the same direction.
If oxygen's unpaired electrons were spinning in opposite directions,
oxygen would be a very reactive radical. So because of that, oxygen itself
is not a very dangerous radical.
But, oxygen can give rise to other active oxygen free radicals like superoxide.
Superoxide is a common oxygen radical. Superoxide is merely an oxygen molecule
to which an electron has been added. This electron may be added by a variety
of ways. A common way in which this may happen is when some reduced metal
-- such as iron or copper -- might donate an electron to oxygen, thereby
making superoxide.
Also, the mitochondria in our cells contain a series of enzymes and other
catalysts known as the respiratory electron transport chain that convert
food components and oxygen into energy and water. However, there are some
components of our respiratory chain that are able to be oxidized and donate
electrons to oxygen to make superoxide.
This respiratory chain should be a nice oxygen cycle and everything should
be fine. The problem arises because there's a slip in the process. Occasionally
an extra electron is added to the oxygen to make it into a free radical;
then there are intermediate steps in the reduction of oxygen that eventually
may lead back to producing water, but along the way we form some potentially
dangerous species.
The superoxide radical is one of the free radicals that is very commonly
produced during metabolism, but there are others.
Passwater: Yes, oxygen forms an unique molecule. Back in what
seems a lifetime ago, during the late 1950's and 1960's -- in my days of
molecular spectroscopy, fluorescence spectrophotometry and quantum mechanics
-- I was intrigued with the fact that most organic compounds exist in nature
in an electronic state called "the singlet state," but because
of oxygen's unique spin characteristics molecular oxygen normally exists
in the "triplet state."
When oxygen absorbs photons of energy and is transformed to the first electronic
state, it can then fairly readily decay into its lowest excited electronic
state, which for oxygen is the singlet state. Where it can persist and do
harm. I want to talk about singlet oxygen with you in more detail later,
and I also want to discuss the role of iron in producing the superoxide
radical, and in the studies linking stored iron in the body with heart disease
and cancer, but for now lets get back to the various types of oxygen radicals.
We hear a lot about the superoxide radical which our body deactivates with
the enzyme, superoxide dismutase. What other oxygen free radicals should
we be concerned with?
Packer: Notice in the oxygen cycle that superoxide
can be converted into a hydroperoxide. Hydroperoxides are not very reactive
unless they are reduced by free iron or copper ions, in which case they
are transformed into hydroxyl radicals. Hydroxyl radicals are quite reactive.
They can be produced at an enormous rate and they persist sufficiently long
to damage proteins, as well as fats. Fats are easy to damage by oxygen-radical
attack. Fats can become free radicals called lipid peroxides (hydroperoxides
of fatty acids). These radicals are not oxygen radicals. Lipid peroxides
can in turn, damage proteins, but hydroxyl radicals, which are oxygen cycle
radicals, can directly damage proteins.
Proteins can be cross-linked together which will impair their functional
activity. Free radical cross-linking damage to DNA may impair its ability
to open up to express genes. Enzyme function would also be impaired.
The body has enzymes which can repair much of the damage to proteins, but
when these enzymes become damaged themselves, repair processes are compromised.
Passwater: How can oxygen radicals do so much damage?
Packer: If a large amount of oxygen radicals and reactive
oxygen species produced by an acute exposure to oxidants occurs, then the
endogenous antioxidant defense mechanisms may be overwhelmed. They will
just simply not be able to cope with a load of free radicals, and this will
initiate free radicals into crucial biological molecules, small molecules
and macromolecules which can be propagated, leading to molecular damage,
cross-linking and inactivation of function activity.
Passwater: What are our defenses against these oxygen radicals?
Packer : These defenses include antioxidant substances and
enzymes specifically designed to quench radicals. These substances, including
vitamin E, ubiquinones (coenzyme Q), carotenoids, thiols such as glutathione,
thiol enzymes, hydroperoxidases and superoxide dismutase (SOD), also have
other functions.
Passwater: How much of these antioxidant nutrients -- vitamins
A, C, E, beta-carotene, selenium and sulfur-containing compounds -- do we
need, and can we get the optimal amount in our diet?
Packer: This is a very difficult question to answer. Obviously,
it depends on lifestyle and on many unanswered questions that are yet to
emerge from epidemiological studies and clinical trials. Nevertheless, the
circumstantial evidence is accumulating rapidly, that higher levels of antioxidants,
certain types at least, have health benefits. Also, keep in mind that there
are dangers because every antioxidant can be a pro-oxidant.
Furthermore, certain nutrients, selenium, for example, may have an optimum
concentration, but its concentration at which it optimally benefits biological
systems is in a narrow range of concentration and if this is exceeded, toxicity
can occur. Hence, we must have an understanding of the amounts and balance
of dietary nutrients.
Passwater: How does vitamin E stop free radical propagation?
Packer: Vitamin E quenches radicals, particularly lipid (fat)
radicals in hydrophobic parts (areas free of water) of our body. This is
usually in lipid membranes or lipoproteins. This is because vitamin E is
only soluble in lipid, and therefore, that is the major place that it can
exert it's protection. It quenches oxygen free-radicals of lipids, such
as lipid peroxyl radicals and lipid alkoxyl radicals. In the process, vitamin
E becomes a free radical itself. One might ask, what does one gain by this?
What one gains is that vitamin E, when it becomes a radical, is less reactive
(a longer living radical), and thus, is not so dangerous to the biological
system. Because it has a longer lifetime, a vitamin E radical can be regenerated
back to normal vitamin E.
Passwater: Vitamin E seems to be a very dynamic molecule.
Packer: This is what I mean by being regenerated. Vitamin
C, for example, can react with a vitamin E radical to regenerate vitamin
E into its natural antioxidant potent form. In this process, vitamin C becomes
a free radical (the semiascorbyl radical). This vitamin C radical can be
regenerated in turn by thiols (a class of sulfur-containing compounds) such
as glutathione. Reduced glutathione -- that is the tripeptide form of glutathione
having an intact sulfhydryl group -- is the cell's primary preventive antioxidant,
present in cells in concentrations as high as one to ten millimolars. When
reduced glutathione neutralizes harmful oxidative radicals, it becomes oxidized
in such a manner that two reduced glutathione molecules are joined together
via a disulfide bond.
It should be noted that vitamin C is present in cells in micromolar quantities
(a thousandth of that of glutathione), and vitamin E in nanomolar (a millionth
of that of glutathione). So there is a hierarchy of interactions between
the various redox-based antioxidants. Chemical reactions occur that lead
to the regeneration, but there are also enzyme-dependent mechanisms and
pathways that we have identified in recent years that are very important
in regenerating vitamin E. So, vitamin E is really a dynamic molecule, which
is in equilibrium with much of the cells of redox metabolism.
Passwater: How do water-soluble antioxidants protect vitamin
E which is fat-soluble?
Packer: Vitamin C appears to be able to react directly with
the vitamin E radical in the membrane, exposed to the surface of membranes
or lipoproteins. Glutathione does not seem to be able to have such a protective
effect, but rather seems to act through keeping vitamin C in its potent
active form (reduced vitamin C). Perhaps figure 3 will help show this inter-relationship.
Passwater: You mentioned vitamin E and vitamin C cycles, how
about beta-carotene, coenzyme Q-10 and thiols?
Packer: Well, as I was saying, there are enzyme-dependent
pathways for regeneration of vitamin E. Coenzyme Q (or ubiquinone 10 or
ubiquinone 50, as it is sometimes designated), which is in natural membranes,
seems to act as a back-up system, reducing vitamin E radicals and thus,
sparing vitamin E and keeping vitamin E efficient. Although the reduced
form of coenzyme Q (ubiquinols) are antioxidants in their own right, they
do not appear to be nearly as effective directly as vitamin E. Hence, this
system of electron transport between coenzyme Q and vitamin E seems to be
important.
Thiols, such as glutathione and alpha-lipoic acid, in their reduced form,
seem to act primarily by regenerating vitamin C. Alpha-lipoic acid is a
somewhat unique naturally-occurring antioxidant in that it is a small with
both water-soluble and lipid-soluble characteristics (dioctanoic acid),
and can directly interact with lipoproteins or membranes to afford protection
by acting as an antioxidant to quench oxygen radicals such as superoxide
and peroxyl radicals.
Passwater: Where is your research leading you now? Do you
believe that we will learn of additional roles and actions for the antioxidant
nutrients?
Packer: Well, we are only seeing the tip of the iceberg because
it is not only understanding how natural antioxidants act, it is being able
to appreciate the interactions that exist between antioxidants. Each antioxidant
does not act on its own, but often antioxidants have interconnected actions,
which determine the antioxidant potency of the body or parts of the body.
In this regard, one area of great interest currently is whether or not carotenoids
such as beta-carotene, directly interact with other lipid antioxidants such
as vitamin E. Vitamin E is a redox-based antioxidant and carotenoids are
not. Do they interact in an additive or synergistic way? In this case, the
answer is not clear. But, in the case of the redox-based antioxidants like
the thiols, the vitamin C system and the vitamin E antioxidant cycle, there
is clearly a synergism that exists when all three components are interacting
with one another. We are pursuing studies with in vivo and in
vitro model systems seeking to elucidate the pathways and potency of
these antioxidant cycles.
Passwater: Thank you for mentioning the synergism of vitamin
E, vitamin C and the thiols. It's my favorite subject. By the way, speaking
of synergism, what supplements do you take?
Packer: I take several supplements including natural carotenoids,
vitamin E, alpha-lipoic acid and vitamin C.
Passwater: Earlier, we discussed the basic oxygen-radical/antioxidant
relationship and its role in health. You mentioned that iron can donate
an electron to oxygen to produce a superoxide radical. Many people are concerned
about the possible implications of a recent Finnish report suggesting that
heart disease may be linked to the amount of iron found in the blood in
the form of ferritin. (1) Many people are concerned that dietary iron can
increase blood ferritin levels, and thus, increase their risk for heart
disease. Some physicians have singled out iron-containing supplements as
being dangerous. In fact, the newspaper reports quoted several physicians
who saw a chance to gang up on all vitamin and mineral supplements.
What do we know about the dangers of the dietary pro-oxidants such as iron?
Packer: Free iron, that is, iron that has been momentarily
liberated from iron-containing proteins can convert mildly reactive oxygen
radicals into highly reactive oxygen radicals, such as hydroxyl radicals.
A research led by Dr. Jukka Salonen of the University of Kuopio recently
reported their study of blood ferritin levels and heart disease incidence
in Circulation [1]. The study is an epidemiological study. Such studies
do not prove anything -- they just verify associations rather causal or
not. And, one such study is in no way definitive.
What intrigues me about the study is that although the association was reported
to be with the amount of ferritin in the blood, there was also mention of
a correlation with dietary iron. However, this data is not presented in
the tables or discussed in further detail.
Passwater: OK, lets hold off the discussion of how dietary
iron may possibly be related to heart disease, and review the background
or iron transport for our readers. As I discuss in my book "Trace elements,
hair analysis and nutrition," iron, in contrast with most other minerals,
is regulated in the body primarily by absorption rather than by excretion.
[2] A number of studies have confirmed the critical importance of the gastrointestinal
tract in controlling the total body iron stores. [3,4,5] Urine contains
very small amounts of iron, and the only iron found in feces is that unabsorbed
from the diet.
Not all forms of iron are absorbed equally. Normally, six to ten percent
of the iron in food is absorbed, but iron-deficient individuals can absorb
more than 15 percent. Persons with iron-deficiency anemia may absorb 50
to 60 percent of the same iron. [2] The iron status of the individual, the
individual's red blood cell production rate, and other factors regulate
the amount of iron transported across the intestinal mucosa.
Packer: Yes, and keep in mind that even in people who eat
a lot of iron, there is not much free iron in the body. People can overload
with iron, but little of the excess iron is found not contained in storage
ferritin. Even in Africans who consume a lot of iron because they cook in
and eat from iron pots, have little free iron in their bodies. They have
abnormal quantities of iron in their livers and spleens stored in organelles
that have developed that even have membranes around them. The body has sequestered
the iron in these deposits.
Passwater: Speaking of peoples from other countries, that
reminds me that there is a significant genetic component to iron storage
-- aside from those having a genetic-defect disease. The control of ferritin
and the plasma-membrane receptor for transferrin is by genetic expression.
The balance between these proteins regulates iron availability, since the
transferrin receptor is required for the uptake of iron, and ferritin is
necessary for storage of any iron temporarily in excess of immediate need.
Packer: It can be expected that a lot of individual variability
in the dynamics and regulation of iron storage occurs in people through
environmental influences and genetic
Passwater: The body produces ferritin, a protein that binds
iron, primarily to store iron in cells. [2] The iron stored in ferritin
is in the form of ferric oxide complex which is surrounded by the apoferritin
protein shell. For every microgram of ferritin in the blood, there is thought
to be about eight milligrams of ferritin stored in cells.
Normally, in healthy adult women, there are 20 to 120 micrograms of ferritin
in each liter of blood, with the "mean" value being 46 micrograms/liter.
For healthy adult men, the range normally is from 30 to 300 micrograms per
liter of blood, with the "mean" value being 127 micrograms/liter.
There is also another compound, called hemosiderin, that functions as an
iron-storage compound.
Iron carried in the bloodstream is mostly carried in a protein called transferrin.
Normally, in the typical healthy adult, there are 200 to 400 milligrams
of transferrin in each deciliter of blood.
Packer: The iron in the body is also incorporated into enzymes,
hemoglobin and myoglobin. But, when these proteins breakdown at the end
part of their normal "lifespan," they release iron into the blood.
Free "reduced" iron is dangerous because it is readily available
to react, therefore, the body goes to great effort to trap free iron ions.
The body binds iron to transferrin and carries it in the blood to the cells
where the iron is transferred to ferritin for storage in the cells.
The potential danger always lies in releasing the iron from proteins. Not
only from transferrin and ferritin, but from the hemoglobin of red blood
cells. The release of iron can occur through tissue injury. As an example,
when you are running on a hard payment, you crush red blood cells every
time your foot hits the ground, usually some iron leaves the red blood cells.
Iron can also be released due to some disease processes.
If you have more free iron around because there is more iron-containing
proteins available to be damaged, then it is logical to expect more iron-catalyzed
free-radical production. Therefore, this report is not illogical, and more
studies should be made.
Passwater: Ferritin was chosen as the risk marker in the Finnish
study because it usually correlates to total iron stores in the body.
However, blood ferritin levels can be raised by inflammation, infection,
chronic disease, thalassemia, liver disease or Hodgkin's disease. So a study
of the association between ferritin and heart disease must determine if
heart disease raises blood ferritin, if some confounding factor increases
both ferritin and heart disease, or if increased ferritin levels increase
the heart disease incidence. An epidemiological study does not determine
which does what, but if additional epidemiological studies support the Finnish
study, much effort should be devoted to laboratory and clinical studies.
Epidemiological studies by themselves are not unlike associating umbrellas
usage with the incidence of rain -- while there is a high correlation between
seeing umbrellas and having rain -- it is not the umbrellas that cause the
rain.
Packer: What we can look at in the laboratory is the role
that oxidative stress can play in releasing iron from its protein carriers.
In a book that I edited, Drs. Barry Halliwell and John Gutteridge discuss
how superoxide radicals liberate iron from ferritin and thus promote lipid
peroxidation. [6] Also, it has been reported that an in vitro study
showed that a combination of physiological concentrations of hydrogen peroxide
and hemin induces rapid peroxidation which releases free iron. There are
other studies supporting a possible synergism between oxidative stress,
free iron and oxidized LDL. Oxidized LDL is now thought to be a major factor
in promoting atherosclerosis.
Passwater: There are other preliminary studies and observations
that seem to fit this thesis. The consensus is that women are protected
from heart disease until after menopause by estrogen. However, as Dr. Jerome
Sullivan of V. A. Medical Center in Charleston has pointed out, this protection
could arise from the fact that women have low iron ferritin due to their
monthly losses.
[7] Neither hypothesis has as yet been well supported and either could be
correct or both may be incorrect.
Support is found in other common observations that could be given different
interpretations to exploit the possible iron link. Aspirin is thought to
reduce heart attacks because it reduces the tendency for blood to clot,
and thus may reduce the probability of clots from blocking the flow in coronary
arteries which is what happens during a heart attack. A new interpretation
could be that aspirin may be effective because it causes gastrointestinal
bleeding, and this blood loss reduces body iron levels.
Some have presented a new interpretation of an old line of thinking to extend
this new observation -- as yet unconfirmed -- to explain a suggested link
between red meat and heart disease -- not by red meat's saturated fat content,
but by its iron content.
Other so-called associations have been reported, but they too are still
weak at this time. As an example, there have been reports that carcinogens
fed to laboratory rats on a high iron diet have increased numbers of tumors.
[8] This is not a test of body stores, nor necessarily of dietary iron.
The effect could be merely due to the fact that ionic iron in the diet reacted
with the carcinogen to make it more potent.
In another study, men with high iron stores were more likely to develop
cancer during the ten years of the study than men with lower iron stores.
However, iron stores were not linked to dietary iron intakes, "which
suggests cancer-prone individuals either exhibit altered absorption or metabolism
of iron." [9] Undoubtedly, this will rapidly become a fertile
area for research. You have already done some research with iron, free-radicals
and heart disease. What have you looked at?
Packer: In studies that we have done in vitro, where
iron is studied during ischemia reperfusion of the isolated animal heart,
iron catalyzes oxygen damage to the heart tissues. However, if we bind the
iron to a sequestering agent in a specific manner, iron is not available
to catalyze oxygen reactions and free radical damage is minimized and this
is accompanied by greater recovery of the hearts in vitro in terms
of mechanical activity (contractility).
Passwater: Singlet oxygen -- an excited electronic state of
oxygen -- contains more energy than the "ground" state or normal
molecular oxygen. When this excess energy of singlet oxygen is discharged,
damage to body molecules can occur. Although this is not free-radical damage,
it is another way in which oxygen can be "our dangerous friend."
Is singlet oxygen a major problem to the body?
Packer: In plants where photosynthesis is occurring, this
is a major problem. The photons of ultraviolet energy are being absorbed
in the process of converting water and carbon dioxide into oxygen and food.
Plants manufacture carotenoids such as beta-carotene to protect against
singlet oxygen by converting it back to normal oxygen.
In the human the action of some enzymes produces singlet oxygen in the dark
regions of the body such as myeloperoxidase of macrophages. However, it's
not known whether this species of activated oxygen is damaging in people.
Passwater: You mentioned that beta-carotene can protect against
singlet oxygen. How about lycopene and vitamin E. Vitamin E can quench singlet
oxygen through an essentially (99%) physical process (Stevens et al, 1974),
is this a meaningful action for vitamin E in the human?
Packer: Vitamin E can quench singlet oxygen, although not
as efficiently as carotenoids -- but since we don't know how significant
singlet oxygen is for human health -- this may be a mute question.
Passwater: Thank you for helping to provide a foundation for
the understanding of antioxidant nutrients, pro-oxidants and free-radical
pathology. I wish our readers can have the opportunity to attend one of
your lectures, as you are the most dynamic scientific lecturer I've ever
witnessed.
REFERENCES
1. High stored iron levels are associated with excess risk ofmyocardial
infarction in Eastern Finnish men.Salonen, Jukka T.; Nyyssonen, Kristiina;Korpela,
Heikki; et al.Circulation 86:803-11 (1992)
2. Trace elements, hair analysis and nutrition.Passwater, Richard A. and
Cranton, Elmer M.Keats Publishing, New Canaan, CT (1983)
3. Bothwell, T. H. and Charlton, R. W.Annu. Rev. Med. 21:145-6 (1970)
4. Forth, W. and Rummel, W.Physiol. Rev. 53:724-92 (1973)
5. Linder, M. C. and Munro, H. N. J. Proc. Fed. Amer. Soc. Exp.Biol. 36:2017-23
(1977)
6. Role of free radicals and catalytic metal ions in humandisease: An overview.Halliwell,
B. and Gutteridge, JMCin: Packer, Lester and Glazer, A. N. (eds)Methods
in Enzymology, vol. 186, pp 1-85, Academic Press,San Diego, (1990)
7. The iron paradigm of ischemic heart disease.Sullivan, Jerome Amer. Heart
J. 17:1177-88 (1989)
8. Iron and the risk of cancer.Stevens, R. G.Med. Oncol. Tumor Pharmacother.
7(2-3):177-81 (1990)
9. Iron: Health-enhancing or cancer promoting?Somer, ElizabethNutr. Rept.
42 (June 1992)
All rights, including electronic and print media, to this article are copyrighted
by © Richard A. Passwater, Ph.D. and Whole Foods magazine (WFC Inc.).
Richard A. Passwater, Ph.D. has been a research biochemist since 1959. His first areas of research was in the development of pharmaceuticals and analytical chemistry. His laboratory research led to his discovery of......more | 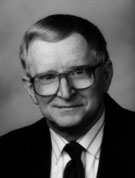 |
|
|
Popular Related Articles/Areas
Popular & Related Products
Popular & Featured Events
Dimensions of Wellness
|
|