| Interviews with Nutritional Experts: Speaking of Radicals: Part II- Reactive Oxygen Species | |
Interview with Dr. William Pryor as interviewed by Richard A. Passwater PhD
Last month, the leading free radical expert, Dr. William Pryor, Boyd
Professor and Director of the Biodynamics Institute at Louisiana State University
at Baton Rouge, used easy to grasp analogies to explain free radicals. This
month, he will discuss common free radicals and other reactive oxygen species
of interest to biochemists. In Part III, he will discuss antioxidant nutrients.
Dr. William A. Pryor is the Director and Boyd Professor of the Biodynamics
Institute of Louisiana State University in Baton Rouge. He has published
over 500 scientific reports and 25 books. He has served as editor of the
leading journal in the field, Free Radical Biology % Medicine and
the book series Free Radicals in Biology.
Passwater: Let's continue with our free radical damage story. What
are some of the common free radicals that we should be concerned about?
Pryor: A common free radical is the hydrogen atom, the simplest free
radical, that's a hydrogen atom (a "H" in chemical symbols) and
a dot (.), so it is written as H.. As I explained earlier, a
free radical has an odd number of electrons, and we indicate this presence
of an odd number of electrons by a dot. Thus, two H. atoms can
combine and form a hydrogen molecule H:H, or H-H. If that hydrogen molecule
were to spit its electrons evenly (homolytically) it would again form the
two hydrogen atom free radicals.
H-H H. + H.
Passwater: How about the oxygen molecule. Isn't the oxygen molecule
a di-radical? The ground state diatomic oxygen molecule has two unpaired
electrons each located in a * antibonding orbital. This helps
give molecular oxygen an interesting array of potential reactivity possibilities.
The oxygen molecule does not have an odd number of electrons, but it does
have two "lone" electrons in separate orbitals and can be thought
of as being two "odd" electrons.
Pryor: Yes, the ground state of the oxygen molecule O2 is a diradical
with two unpaired electrons ( .O-O.). Singlet oxygen,
which is excited by 23 kcal/mol , has all paired electrons (O=O).
This leads us to another free radical that is very common in biology, the
superoxide radical (the superoxide radical anion). It is formed by the one
electron reduction of a dioxygen molecule. Reduction is the opposite of
oxidation, and occurs when an atom or molecule accepts one or more electrons.
So we have O2 -- a dioxygen molecule -- adding an electron and the electron's
negative charge, giving the superoxide anion. Thus, the superoxide anion
radical is written as O2.-. (The convention says that the dot
precedes the minus and both are written as superscripts above the oxygen.
I love the fact that the dot precedes the minus -- radicals are more important
than ions even in the people who just name things.)
Passwater: I understand your pleasure in seeing that at last proper
recognition is coming to proper free radical terminology, but conventional
terminology still doesn't recognize the free radical nature of the oxygen
atom. As we just discussed, the oxygen molecule is a di-radical, and thus
it should be written as O2.., with the two dots representing
lone non-paired electrons.
Pryor: Technically, you are right, but it would be too confusing
for beginning chemistry students to have to contend with oxygen as a di-radical
rather than a simple molecule. But, I wouldn't mind seeing the oxygen molecule
written as O2 ( ) when free radical chemistry is the focus.
Passwater: OK, I'm sorry I interrupted your response, let's go back
to the superoxide anion.
Pryor: I was about to say that the superoxide anion is frequently
formed and the reason it is formed in biological systems is because we use
oxygen to oxidize our food and make energy for us to live. This happens
in the mitochondria present in the cytoplasm of most living cells. However,
very frequently instead of food oxidation in mitochondria happening in two
electron steps, about one to two percent of the time, it occurs in a single
electron step producing the superoxide anion radical.
Oxygen can be reduced to superoxide radical anions. In the body,
the mitochondria use oxygen to metabolize food to produce energy. Water
and carbon dioxide are formed directly in this major process. Outside of the body, processes such as photosynthesis
again free the oxygen from the water and carbon dioxide. However, some of
the oxygen is converted into superoxide radical anions as explained in the
interview with Dr. Pryor. This minor pathway is shown by the arrow in the
clockwise direction.
Once you get a superoxide radical you are going to have radicals propagating
damage throughout the biological system until you have a termination --
until there is another radical species that can couple and somehow quench
that superoxide. In biology that can be done, for example, by superoxide
dismutase (SOD), the enzyme whose purpose is to control superoxide levels.
SOD can take two superoxide molecules -- therefore two free radicals --
and dismutate them -- that is, take an electron from one and add it to the
other. When an electron is taken from superoxide, it then becomes a dioxygen
molecule again. So one of the products of dismutation is the dioxygen molecule.
When SOD adds an electron to the other superoxide molecule, it goes from
02.- to 02= and we no longer have a dot because we
have pairs of electrons and 02= would pick up two protons and
become hydrogen peroxide, which itself could be a damaging species in biology.
Superoxide Dismutase (SOD) converts the superoxide radical
anion back to oxygen. The next step in the oxygen cycle is the production of hydrogen
peroxide as indicated by the arrow continuing in the clockwise direction.
Passwater: This is the oxygen cycle converting food to carbon dioxide
and water. You have taken us to hydrogen peroxide. Fortunately, just as
our bodies can produce the antioxidant enzyme, superoxide dismutase (SOD),
which can dismutate some or even most of the superoxide formed, depending
on our efficiency at making SOD, our bodies can also make the antioxidant
enzyme, catalase, to convert some of the hydrogen peroxide into water. What
next?
Pryor: Hydrogen peroxide can add another electron and split at the
0-0 bond giving HO. and HO-. That is, you get a negative charged
species and a free radical. The free radical you get is called the hydroxyl
free radical, HO. , an extremely reactive free radical. It reacts
with almost everything as fast as it collides with things. So in other words
HO. reacts almost every time it bumps into another molecule,
generally to pull off a hydrogen atom and make water and also produce another
free radical in the organic residue (the remaining part of the molecule).
The next step in the oxygen cycle can be the conversion of hydrogen
peroxide into the highly reactive hydroxyl radical.
Now if you took that hydroxyl free radical and added still another electron
you would get HO- and if that picked up a proton it would become water.
So usually we burn food and we make water and release lots of energy:
4e- 4H+
O2 2H2O + energy
However, On occasion, the mitochondrion goes through one electron steps
leading in sequence to superoxide, hydrogen peroxide, hydroxyl radical,
and finally water.
e- e- 2H+ e- e- H+
O2 O2.- O2= H2O2 HO- + HO. H2O
Passwater: We often use the term "Reactive Oxygen Species"
(ROS) now to include species other than radicals. How about singlet oxygen?
You mentioned that singlet oxygen has all paired electrons and is not a
free radical, but it can damage body components.
What happens when the energy of sunlight or chemical reactions in the body
transfer just enough energy to oxygen to raise the oxygen ground state to
the first excited triplet state, and via internal conversion, the excited
triplet state relaxes into the singlet state by reversing the spin of the
lone electron, which yields a long-lived species having excess energy?
Pryor: I do not think singlet oxygen is important in any organ except
those that are irradiated directly, such as the eye and the skin.
Passwater: Let's return to your interrupted dancing representation
of free radical propagation for a moment. The odd dancers that are created
represent damage to other molecules in the body. As an example, we can have
lipid peroxidation. Please tell us a little about the chemistry of the peroxyl
radical.
Pryor: The peroxyl radical actually is reasonably stable. I've calculated
a lifetime of seconds for peroxyl radicals.
Passwater: How about transition metals and their role in biochemistry.
Their odd electrons are not in their outermost electron shell. Do transition
metals participate in one-electron transfer reactions. Do transition metals
really contribute to free radical production in the human body or is this
just something we are just starting to look at?
Pryor: Metals are very important in the damage that radicals produce
and the subject has been studied by a large number of very good scientists,
but it is still very murky. Transition metals that have two oxidation states
that differ by one electron oxidation can cause peroxides to decompose.
For example, iron and copper ions can cause peroxides to decompose and can
themselves go up to the next higher oxidation state -- as an example ferrous
goes to ferric and cuprous to cupric. Exactly how this works in a biological
system is not clear, because there are no free metal ions in biology, they
are all complexed to proteins. As an example, iron is carried by hemoglobin,
transferrin and ferritin. Incidentally iron is stored in ferritin as ferric
iron and superoxide will reduce those ferric ions to ferrous ions and ferrous
ions are then released by the ferritin. Some scientists feel this is a critical
change that superoxide causes that can release iron to be available to catalyze
radical reactions.
We have been studying the cigarette tar free radical which is a very stable
radical that has an infinite lifetime. It has the property, like superoxide,
of being able to reduce the ferric ions in ferritin to produce ferrous and
cause iron to be released from ferritin. In this case, although you could
have the cigarette tar semiquinone radical reduce oxygen to make superoxide
and superoxide then cause the reduction of ferric ions, it actually is the
cigarette tar free radical itself that is the culprit. The cigarette tar
free radical, and there can be
5 x 1014 in each puff of smoke inhaled, reduces the iron in ferritin,
rather than reducing oxygen. Thus, once you have odd electron species, you
can get a cascade of changes with many branching points. And each of these
branches of free radicals may produce their own type of biological damage.
Passwater: Well that's the first half of the story. I am sure our
readers will want to hear your explanation in Part III of how antioxidant
nutrients protect us against these deleterious free radicals. Your analogies
of these various categories of defenders as different components of the
body's army -- the foot soldiers, the artillery and the assault troops make
it easier to understand the roles of each of these synergistic defenders.
Thank you Dr. Pryor.
All rights, including electronic and print media, are copyrighted to Richard
A.Passwater, Ph.D. and Whole Foods magazine (WFC Inc.).
Richard A. Passwater, Ph.D. has been a research biochemist since 1959. His first areas of research was in the development of pharmaceuticals and analytical chemistry. His laboratory research led to his discovery of......more | 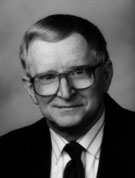 |
|
|
Popular Related Articles/Areas
Popular & Related Products
Popular & Featured Events
Dimensions of Wellness
|
|